Thank you for visiting nature.com. You are using a browser version with limited support for CSS. To obtain the best experience, we recommend you use a more up to date browser (or turn off compatibility mode in Internet Explorer). In the meantime, to ensure continued support, we are displaying the site without styles and JavaScript.
Scientific Reports volume 13, Article number: 2683 (2023 ) Cite this article Dandelion Extract

The members of the genus Phyllanthus have long been used in the treatment of a broad spectrum of diseases. They exhibited antiproliferative activity against various human cancer cell lines. Breast cancer is the most diagnosed cancer and a leading cause of cancer death among women. Doxorubicin (DOX) is an anticancer agent used to treat breast cancer despite its significant cardiotoxicity along with resistance development. Therefore, this study was designed to assess the potential cytotoxicity of P. niruri extracts (and fractions) alone and in combination with DOX against naïve (MCF-7) and doxorubicin-resistant breast cancer cell lines (MCF-7ADR). The methylene chloride fraction (CH2Cl2) showed the most cytotoxic activity among all tested fractions. Interestingly, the CH2Cl2-fraction was more cytotoxic against MCF-7ADR than MCF-7 at 100 µg/mL. At sub-cytotoxic concentrations, this fraction enhanced the cytotoxic effect of DOX against the both cell lines under investigation (IC50 values of 0.054 µg/mL and 0.14 µg/mL vs. 0.2 µg/mL for DOX alone against MCF-7) and (1.2 µg/mL and 0.23 µg/mL vs. 9.9 µg/mL for DOX alone against MCF-7ADR), respectively. Further, TLC fractionation showed that B2 subfraction in equitoxic combination with DOX exerted a powerful synergism (IC50 values of 0.03 µg/mL vs. 9.9 µg/mL for DOX alone) within MCF-7ADR. Untargeted metabolite profiling of the crude methanolic extract (MeOH) and CH2Cl2 fraction exhibiting potential cytotoxicity was conducted using liquid chromatography diode array detector-quadrupole time-of-flight mass spectrometry (LC-DAD-QTOF). Further studies are needed to separate the active compounds from the CH2Cl2 fraction and elucidate their mechanism(s) of action.
Several challenges for cancer treatment might result in treatment failure such as, acquired drug resistance which results in the decrease in the intracellular drug concentrations with limited cancer proliferation inhibition and ultimately metastasis1. Accordingly, different dose regimens, higher chemotherapeutic doses or prolonged treatment might be needed to achieve similar efficacy which will lead to more adverse effects2. Doxorubicin (DOX), is the standard first-line adjuvant chemotherapy in the treatment of solid tumors of disparate origins especially, early and metastatic breast cancer, one of the most common female malignant neoplasms3,4. Despite showing promising clinical results, there are clinical limitations arising from its vulnerability to the development of multidrug resistance (MDR), possibly due to the overexpression of ATP binding cassette transporters as well as developing debilitating side effects hurdle against dose escalation and completion of treatment course5,6. Cardiotoxicity and other multi-organ toxicities such as liver are the major limitation for doxorubicin treatment continuation and dose escalation and accordingly, result in restricted anticancer efficacy7,8.
Therefore, there is a necessity for a proper approach to potentiate the DOX chemotherapeutic efficacy and limit its side effects by reducing its doses. It is worth mentioning that cancer cells typically display much higher ROS levels which counteract the activity of several cytotoxic agents such as DOX9.
Yet, cancer cells are naturally more dependent on antioxidant systems and ROS scavenging metabolic pathways. Consequently, impairing antioxidant system may be a potential therapeutic approach against cancer10.
To this aim, natural products are gaining prominent attention in drug discovery, especially for the development of anticancer drugs. Several plant extracts and bioactive compounds demonstrated promising anticancer properties as well as chemomodulatory effects11,12.
Genus Phyllanthus is one of the largest genus in the family Phyllanthaceae having 11 sub-genera compromising over 700 well-known species and are globally disseminated in the tropics and subtropics13. Phyllanthus niruri L. (Syn P. amarus Schum & Thonn) is a traditional pharmaceutical plant that has been described for its various medicinal activities such as hepatoprotective, antibacterial, antiviral, anti-diabetes, anti-obesity, antihyperlipidemic, and anti-inflammatory14,15,16,17.
In recent years, Phyllanthus species are examples of promising sources of natural anticancer products18,19. P. niruri demonstrated anticancer properties against lung cancer20, breast cancer21, prostate cancer22, and hepatocellular carcinoma23 . Thanks to its antiproliferative effect on cancer cells by modulating various cell signaling pathways, reports showed its advantage of being safe on normal cells23,24. Yet, its efficacy against high recurrent and resistant cancer such as breast cancer is still unclear.
In our previous work, we prepared lignan-rich extract from the aerial parts of P. niruri using nonconventional methods to increase the level of lignans calculated as phyllanthin25. Herein, we investigated the potential cytotoxicity of the different extracts from P. niruri and studied the influence of their combination with DOX on the cytotoxic profile of DOX in naïve and doxorubicin-resistant breast cancer cell lines.
Phyllanthin was purchased from Fluka (Lot # BCBL2476V, product of India). The analytical grade solvents used in the extraction and chromatographic separation were purchased from El Gomhouria for Drugs Co. (Cairo, Egypt). Precoated TLC plates (20 × 20 cm), silica gel F254 and RP silica gel were obtained from Sigma-Aldrich chemicals (Darmstadt, Germany). Sulforhodamine-B (SRB) and doxorubicin were purchased from Sigma Chemical Co. (St. Louis, MO, USA). Acetonitrile, methanol, and formic acid used (HPLC grade) were obtained from Fisher Scientific, Waltham, MA, USA. Water was purified using a Milli-Q system (Millipore, Bedford, MA, USA).
Samples of aerial parts of P. niruri L. were collected in August 2018, from Tasek Gelugor, Penang, Malaysia and the collection procedure was in compliance with the national and international guidelines and legislation. The plant materials were supplied and identified by the staff members of the Malaysian Agricultural Research and Development Institute (MARDI) through Natural Wellness Co., Cairo, Egypt. A voucher specimen (PN-01-082018B) was kept at the herbarium of the Faculty of Pharmacy, Cairo University.
The powdered aerial parts of P. niruri (750 g) were exhaustively extracted with MeOH (3 × 1.5 L) and another amount (100 g) was extracted with boiling distilled water (2 × 150 mL) using homogenizer (3 times each). The solvent was removed under vacuum, at a temperature not exceeding 60 °C to yield 93 g of MeOH extract (Ext-1) and 16 g of water extract (Ext-2). Part of the MeOH extract (70 g) was suspended in H2O (600 mL) and extracted with CH2Cl2 (3 × 400 mL). The solvent was removed by distillation to yield CH2Cl2 fr. (Fr-3, 24.5 g). The aqueous remaining fraction (44 g) was passed through Diaion-HP20 (4 × 20 cm) and eluted with 100% water (500 mL), 50% MeOH (500 mL) followed with 100% MeOH (750 mL) to give Fr-4 (26.2 g), Fr-5 (13.2 g), and Fr-6 (4.1 g), respectively. The resulting MeOH extract and CH2Cl2 fraction were analyzed by Liquid chromatography diode array detector-quadrupole time-of-flight mass spectrometry (LC-DAD-QTOF) and were biologically tested.
The CH2Cl2 fr. (10% solution in CH2Cl2) was subjected to preparative TLC (solvent system: n-hexane-ethyl acetate, 2:1) on silica gel plates (Silica gel 60, Merck; 0.5 mm thick, 20 × 20 cm). Each plate was divided into 4 bands (B1–B4) and each band was scratched, eluted with a mixture of CH2Cl2-MeOH (1:1), and evaporated and the the samples kept at 4 °C till use.
Samples from the MeOH extract and CH2Cl2 fraction were prepared in MeOH (LC–MS grade) in concentration of 50 mg/mL. The solution was then filtered and stored in sealed glass vials until analysis.
The liquid chromatographic system was an Agilent Series 1290 and the separation was achieved on an Acquity UPLC™ HSS C18 column (100 mm × 2.1 mm I.D., 1.8 µm). The mobile phase consisted of water with 0.1% formic acid (A) and acetonitrile with 0.1% formic acid (B) at a flow rate of 0.21 mL/min. The analysis was performed using the following gradient elution: 95% A/5% B to 35%B in 15 min, in next 5 min to 70%B and finally to 100% B in next 2 min. A 3 min wash followed each run with 100% B and an equilibration period of 5 min with 95% A/5% B. One microliter of the sample was injected. The column temperature was 40 °C.
The mass spectrometric analysis was performed with a QTOF-MS–MS (Model #G6545B, Agilent Technologies, Santa Clara, CA, USA) equipped with an ESI source using the following parameters: drying gas (N2) flow rate, 13 L/min; drying gas temperature, 300 °C; nebulizer pressure, 30 psig, sheath gas temperature, 400 °C; sheath gas flow, 12 L/min; capillary voltage, 4000 V; nozzle voltage, 0 V; skimmer, 65 V; Oct RF V, 750 V; and fragmentor voltage, 150 V. All the operations, acquisition and analysis of data were controlled by Agilent MassHunter Acquisition Software Ver. A.10.1 and processed with MassHunter Qualitative Analysis software Ver. B.10.00. Each sample was analyzed in positive and negative modes over the range of m/z = 50–1100 and extended dynamic range (flight time to m/z 1700 at 2 GHz acquisition rate). Accurate mass measurements were obtained by means of reference ion correction using reference masses at m/z 121.0509 (protonated purine) and 922.0098 [protonated hexakis (1H, 1H, 3H-tetrafluoropropoxy) phosphazine or HP-921] in positive ion mode, while at m/z 112.9856 (deprotonated trifluoroacetic acid-TFA) and 1033.9881 (TFA adducted HP-921) were used in negative ion mode. Samples were analyzed in all-ion MS–MS mode, where experiment 1 was carried out with collision energy of zero and experiment two with a fixed collision energy of 45 eV.
Human breast cancer cell line (MCF-7), doxorubicin-resistant breast cancer cell line (MCF-7ADR), rat normal cardiomyocytes (H9C2) and mouse normal hepatocytes (BNL) were obtained from Nawah Scientific Inc. (Mokatam, Cairo, Egypt) and cultured in DMEM (Dulbecco’s modified eagle’s medium), Gibco, USA. Fetal bovine serum (FBS) at a concentration of 10% and 100 units/mL of penicillin/streptomycin (PS) were added to the culture media. The cells were incubated at 37 °C in a humid environment that contained 5% CO2.
The cell viability was determined following the method reported by Skehan et al.26. MCF-7, MCF7ADR, H9C2 and BNL cells were seeded in 96‐well plates, approximately 105/well. After treatment of DOX and/or P. niruri fractions and subfractions with concentrations of 0.01, 0.1, 1, 10 and 100 ug/ml for 72 h. We replaced the media with 150 μL of 10% TCA (trichloroacetic acid) (Merck) for 1 h at 4 °C, followed by 5 timed washing with distilled water. After that, we added 70 μL of 0.4% w/v SRB solution (Sigma‐Aldrich) at room temperature for ten minutes in the dark. Cells were washed with 1% acetic acid (Merck) 3 times and allowed to a 24 h air-dry. Then, we added 150 μL of Tris Base (10 mM) (Merck) and the O.D. was determined at 540 nm by a microplate reader (FluoStar Omega, BMG Labtec, Ortenberg, Germany).
MCF-7ADR cells were treated with DOX and B2 alone or combined at their pre-determined IC50 values for 48 h. After treatment, cells were washed with PBS, trypsinized, centrifuged, and pellets were lysed using 0.5 ml of ice-cold lysis buffer (Cat No: FNN001; Invitrogen). The intracellular GSH levels were determined using the commercially available colorimetric kit to determine the GSH content (Biodiagnostics Company, Cairo, Egypt) according to the manufacturer’s instructions27. The total protein content in the cell lysate was determined using Bradford method for normalization.
The dose–response curves of compounds were investigated applying the Emax model as previously described28. The combination index (CI) was calculated as previously described29. Drug interactions are classified as additive if CI is between 0.8 and 1.2, antagonistic if CI is > 1.2, and synergistic if CI is < 0.8. All data are presented as means ± SD (n = 3). Analysis of variance (ANOVA) with Tukey’s post-hoc test (P < 0.05) was applied using GraphPad Prism Software version 6.
The metabolite profiling of the methanol extract (MeOH) and the methylene chloride fraction (CH2Cl2) with potential cytotoxicity was analysed using liquid chromatography diode array detector-quadrupole time-of-flight mass spectrometry (LC-DAD-QToF). Figures 1 and 2 showed the base peak chromatograms (BPC) of the MeOH and the active CH2Cl2 fraction in negative and positive modes. Adopted analytical setup in both ESI modes led to the identification of 53 in both extracts with low abundance of metabolites 5, 9–14, within 30 min (Table 1). The metabolites were tentatively characterized based on their UV spectra (254, 280 and 330 nm), retention time, accurate mass, and MS fragmentation patterns and by comparing their mass spectra with those reported in the phytochemical dictionary of natural products database (CRC) and from the reported literature30,31,32,33.
LC-QToF total Ion Chromatograms (TOC) of the CH2Cl2 and MeOH extract of P. niruri: in negative (A, B) and positive modes and (C, D).
LC-DAD chromatograms of the CH2Cl2 fraction (A–C) and MeOH extract (D–F) of P. niruri. at 254, 280 and 330 nm.
The identified compounds are represented by 42 phenolic acids and phenolic derivatives, 7 fatty acids, an amino acid, a sugar derivative, an alkaloid, and a dicarboxylic (Fig. 3 and Table 1). Most of peaks belonging to acids and phenolics were observed in negative ion mode (Table 1) showing [M–H]− ions with the loss of one mass unit, except for compound 5 which was observed in positive mode. Details of metabolites assignment will be discussed in the coming section.
Examples of structure of some compound identified in P. nirri.
Sulforhodamine B (SRB) assay was used to assess the potential cytotoxicity of six extracts of P. niruri, including total methanol (Ext-1), aqueous (Ext-2), CH2Cl2 (Fr-3), and diaion fractions (Fr-4: water, Fr-5: 50% MeOH and Fr-6: 100% MeOH), against naïve breast cancer cell line (MCF-7) and doxorubicin-resistant breast cancer cell line (MCF-7ADR).
All the tested fractions except for Fr-3 did not show good cytotoxicity against the two cell lines since their IC50 values were higher than 100 μg/mL (Table 2). On the other hand, Fr-3 showed substantial cytotoxicity against MCF-7 and MCF-7ADR cells with IC50 values of 97.2 ± 1.13 μg/mL and 51.1 ± 0.93 μg/mL, respectively. Interestingly, MCF-7ADR cells (Dox-resistant) were more sensitive than MCF-7 cells (Naïve) to Fr-3 treatment (Table 2).
To study the outcome of P. niruri on the cytotoxic profile of DOX in MCF-7 and MCF-7ADR cells, we assessed the dose–response curves of DOX alone and in combination with the different extracts of P. niruri (Fig. 1S-A, B) (Table 3).
The chemomodulatory effect of P. niruri extracts and fractions at noncytotoxic concentrations were studied along with serial concentrations of DOX within breast cancer cells using SRB assay as described in the previous section.
In MCF-7 cells, DOX exerted a dose-dependent cytotoxic activity, and the viability started to decrease markedly at a concentration of 0.1 µg/mL with an IC50 value of 0.2 ± 0.06 µg/mL (Fig. 1S-A). Except for Fr-3, all tested extracts, and fractions of P. niruri (Ext-1, Ext-2, Fr-4, Fr-5, and Fr-6) at a sub-toxic concentration (100 µg/mL) in combination with DOX did not improve the cytotoxic/antiproliferative effect of DOX against MCF-7 cells. This can be observed from the increase of IC50 values of DOX to ~ 10 times after combination with the different extracts. Conversely, the combination of DOX with a sub-cytotoxic concentration of Fr-3 (10 µg/mL), significantly increased the antiproliferative/cytotoxic effect of DOX against MCF-7 cells; the IC50 values of DOX were 0.2 ± 0.06 µg/mL and 0.054 ± 0.001 alone and in combination with Fr-3, respectively (Fig. 1S-A). A similar result was observed for the combination of DOX with Fr-3 at higher concentration (100 µg/mL) and IC50 value were decreased to 0.014 ± 0.002 µg/mL (1S-A).
Similarly, in MCF-7ADR cells, DOX also showed gradient cytotoxic activity with increasing concentration; viability started to drop significantly at a concentration of 1 μg/ml with an IC50 value of 9.9 ± 1.1 µg/mL (Fig. 1S-B). Unlike the MCF-7 cell line, combining Ext-1, Fr-5, and Fr-6 with DOX significantly improved the cytotoxic profile of DOX in MCF-7ADR cells, decreasing both the resistant fraction and IC50 of DOX to 0.27 ± 0.01 μg/mL, 1.9 ± 0.45 μg/mL and 3.3 ± 0.07 μg/mL, respectively. Additionally, a more improvement in the cytotoxic profile of DOX was observed after the combination with Fr-3 (10 μg/mL), decreasing IC50 of DOX to 0.23 ± 0.01 μg/mL along with abolishing the resistant fraction. On the other hand, the combination of Fr-4 with DOX did not significantly influence the cytotoxic/antiproliferative activity of DOX against MCF-7ADR cells.
The results of these two cell lines can declare that Fr-3 possessed the most promising chemomodulatory effect for DOX in breast cancer cells. Therefore, additional subfractions from Fr-3 were prepared and assessed for further cytotoxic and chemomodulatory potential.
Four TLC subfractions from fr-3 (CH2Cl2) were obtained by preparative TLC and named B1, B2, B3, and B4. In MCF-7cells, all the tested subfractions didn’t show observable cell-killing effects even until 100 μg/mL concentration. Conversely, in MCF-7ADR cells, all subfractions showed cell-killing effects at a concentration of 100 μg/mL with calculated IC50 values of 188.6 ± 2.3 μg/mL, 86.4 ± 1.5 μg/mL, 100.4 ± 1.8 μg/mL and 112.7 ± 2 μg/ml for B1, B2, B3 and B4 respectively (Table 4). It is worth mentioning that MCF-7ADR cells were more sensitive to treatment with these subfractions. Accordingly, it was selected to further investigate the chemomodulatory effect of these subfractions on DOX. Combinations of the four subfractions (B1–4) with DOX were clearly synergistic with very low CI-values of 0.157, 0.003, 0.038, 0.061, respectively (Table 5). Based on these CI-values, B2 was the most promising fraction that significantly reduced the IC50 of DOX from 9.9 ± 1.1 to 0.03 ± 0.001 and abolished the resistance fraction from 5.2 ± 0.04 to nearly zero (Table 5). It merits mentioning that subfractions combinations with DOX drastically improved the cytotoxic profile of DOX against MCF-7ADR (Fig. 2S).
The most promising cytotoxic fraction (B2) was further assessed for its possible cardiotoxic and/or hepatotoxic effects. H9C2 (normal cardiomyocyte) and BNL (normal hepatocytes) were incubated with B2 for 72 h, and cell viability was assessed by SRB and compared with the cell viability of DOX (Fig. 3S).
Dox inhibited cell proliferation of both H9C2 and BNL cells in a dose-dependent manner, with IC50 values of 0.0153 ± 0.001 µg/ml and 0.0571 ± 0.002 µg/ml, respectively. While B2-fraction did not affect the cell viability of both H9C2 and BNL cells up to a concentration of 100 µg/ml, indicating the safety of B2 fraction on heart and liver normal cells in contrast to DOX (Fig. 3S).
In this study, we tested the influence of the most potent cytotoxic fraction (B2) and doxorubicin alone or in combination on the intracellular level of GSH within MCF-7ADR cells. Numerous studies have indicated GSH activity alterations after treatment with doxorubicin in vitro. Herein, it was found that DOX treatment resulted in a significant increase in GSH levels (3.81 ± 0.2 µmol/mg protein) compared to non-treated cells (2.71 ± 0.1 µmol/mg protein). On the other hand, B2 alone showed a significant reduction in GSH level to 1.67 ± 0.5 µmol/mg protein compared to non-treated cells (2.71 ± 0.1 µmol/mg protein). The combination of B2-fraction and DOX normalized the intracellular GSH level and brought back to be non-significant from untreated cells (Fig. 4).
Effects of treatment with IC50 values of B2-fraction, DOX alone or their combination on the intracellular level of GSH within MCF-7ADR cells. Data are presented as mean ± SD; n = 3. *Significantly different from DOX treatment. **Significantly different from control.
Untargeted metabolite profiling of the crude MeOH extract and the most active CH2Cl2 fraction with potential cytotoxicity was conducted using RP‐LC‐DAD‐ESI‐QTOF‐MS. The results of the analysis revealed the identification of 53 in both extracts with different abundance using both ESI modes (Table 1). The metabolites were tentatively characterized based on their UV spectra (254, 280 and 330 nm), retention time, molecular weight, MS fragmentation patterns and comparing their mass spectra with those reported in the phytochemical dictionary of natural products database (CRC) and reported literature30,31,32,33.
The identified metabolites (Fig. 3, Table 1) are belonging to different phytochemical classes, such as phenolic acids and phenolic derivatives (14), ellagitannins (12), flavonoids (10), fatty acids (7), lignans (6), and amino acid (1), sugar derivative (1), alkaloid (1), and dicarboxylic (1). Details of metabolites assignment will be discussed in the coming section.
Phenolic, phenolic acids, alkaloid, ellagitannins and flavonoid glycosides are eluted first in the chromatogram followed by fatty acids, flavonoid aglycones, while the lignans, and were emerged by end of the chromatogram. Most of peaks belonging to acids and phenolics compounds were observed in negative ion mode (Table 1).
Among the fifty-three identified metabolites, sixteen were reported in P. niruri for the first time and the metabolites identified were 1, 4, 5, 7, 8, 10, 30, 35, 36, 41–47. The rest of the metabolites are either previously identified in P. niruri or from other Phyllanthus species. The newly identified metabolites in P. niruri and in genus Phyllanthus are classified into sialic acid derivative (1), phenolics and phenolic acid derivatives (4, 7, 8, 10, and 30), organic acid (35), a flavonoid (36), polyketide alkaloid (5), and fatty acids (41–47).
Compound 1 was identified as 2-deoxy-2,3-dehydro-N-acetylneuraminic acid (sialic acid derivative) by observing mass ions at 290.0882 [M-H]-, and fragments at m/z 200.0567 [M-H-C3H6O3]-, 128.0356 [M-H-C3H6O3-C3H4O2]-. This compound was previously identified in Nymphaea nouchali stem34, and detected in edible bird’s nest, a nutrient-rich salivary bioproduct produced by swiftlets in Southeast Asia35.
Compound 4 was identified as vanillic acid 4-sulfate, previously isolated from seaweeds Sargassum sp., Centroceras sp., Ulva sp.36, and detected in the urine of healthy rats supplemented for 35 days with cranberry extract37. It showed a molecular ion peak [M–H]− at m/z 290.0882, in addition to the fragmentation pattern with ions at m/z 203.0019 [M–H–CO2]−, 121.0296 [M–H–CO2–SH2O3]−, 108.0220 [M–H–CO2–SH2O3–CH]−, 96.9604 [H2SO4]−, 80.9656 [H2SO3]−38.
Compound 5 was identified as sesbanimide A based on the fragmentation pattern with ions at m/z 328.1399 [M + H]+, 310.1290 [M + H–H2O]+, 250.1655 [M + H–H2O–C2H4O2]+, 232.1550 [M + H–2H2O–C2H4O2]+, 132.0811 [M + H–2H2O–C2H4O2–C4H4O3]+, 120.0810 [M + H–2H2O–C2H4O2–C5H4O3]+. This compound was detected and isolated from two marine alphaproteobacteria Stappia indica PHM037 and Labrenzia aggregata PHM03839, in Wu-Ling-San traditional Chinese formula40, and from Sesbania drummondii seed41.
Compounds 7 and 8 had deprotonated molecular ions [M–H]− at m/z 371.0619 and 357.0826, and molecular formulae C15H16O11 and C15H18O10, respectively. They were tentatively identified as caffeoylglucaric acid isomer (7) isolated from edible wild calafate berry (Berberis microphylla G. Forst)42 and dihydrocaffeic acid 3-O-glucuronide (8) detected among the polyphenol-derived metabolites in human plasma after coffee consumption43,44,45. The identification of compound 7 as caffeoylglucaric acid isomer was achieved through the presence of the fragment ions at m/z 315, 209 and 191. According the reported literature42, the fragment at m/z 209 can be interpreted as an aldaric acid moiety like glucaric, with subsequent loss of H2O, to give rise the fragment ion at m/z 191 [209 − H2O]−. This compound was tentatively characterized to be caffeoylglucaric acid isomers, in agreement with the profiles previously reported for B. microphylla G. Forst and B. thunbergii DC46. Compound 8 was tentatively identified as dihydrocaffeic acid 3-O-glucuronide with [M − H]- m/z at 357.0826, and further confirmed from ions at m/z 181 due to loss of glucuronide from parent ion45.
P. niruri showed the presence of compound 10 for the first time, it showed a molecular ion peak [M + H]− at m/z 623.0883 corresponding the molecular formula C26H24O18 with exact mass of 624.0963 and tentatively identified as dihydrovirganin. In addition, it showed the loss of C9H8O8 fragment at m/z 355.0665 [M–H–C9H8O8]−, and 234.0405 [M–H–C9H8O8–C7H5O2]−. The closely related virganin metabolite (C26H22O18) was identified in P. niruri47, P. virgatus48, and in P. urinaria and P. amarus49.
The metabolite 30 was tentatively characterized as elagitannin 4-O-brevifolincarbonyl- 1-O-galloyl- 3,6-O-hexahydroxydiphenoyl-D-glucopyranose50 with a molecular formula C40H28O25 and exact mass of m/z 908.0920. It showed the presence of [M–H]− at m/z at 907.0847 and fragments of m/z at 611.0708, and 169.0141.
The metabolite 35 was tentatively identified as azelaic acid, a dicarboxylic acid had the molecular formula C9H16O4 and exact mass of 18.0974. Azelaic acid produced m/z 187.0974 [M–H]− as the ion base peak in negative ion mode51,52. Azelaic acid is an organic acid compound observed in wheat, rice, rye and barley and it has antimicrobial and anti-inflammatory properties, which make it effective in the treatment of skin conditions like acne and rosacea51.
Compound 36 was observed only in negative ion mode and was identified as 3,4,5,7-Tetrahydroxyflavone-8-sulfonic acid (luteolin-8-sulfonic acid), a flavone sulfonic acid had the molecular formula of C15H10O9S and exact mass of 366.0046 and m/z 364.9973 [M–H]−. It showed mass ion m/z 285.0403 [M–H–SO3]− corresponding to the loss of a sulfonyl group.
Peaks from 41 to 43 were identified as fatty acid isomers. They had deprotonated molecular ions [M–H]− at m/z 327.2175, and fragments ions at m/z 285.0797, 215.1289, 171.1027, 85.0299. They were identified as trihydroxy-octadecadienoic acid isomers. On the other hand, the peaks from 44 to 47 were identified as fatty acid isomers of trihydroxy-octadecanoic acid. They had deprotonated molecular ions [M–H]− at m/z 329.2332.
Six lignans were characterized in the current study, while only four were nirtetralin, phyllanthin, hypophyllanthin, and niranthin, were previously confirmed from our previous work25.
Breast cancer continues to be a major global health problem and it possess high incidence rate in women with subsequent very high rates of morbidity and mortality53,54. Doxorubicin (DOX) is one of the mostly used chemotherapeutic medications for breast cancer. While DOX treatment initially causes clinical responses, its longstanding success may be halted due to the emergence of drug toxicities and resistance55. Currently, combining the natural products with chemotherapeutic agents has drawn researchers’ attention as it was observed to enhance the effectiveness of conventional chemotherapeutic medications and/or safeguard patients from their adverse effects56.
Therefore, in this study, we explored the potential cytotoxic activity of P. niruri, a well-established bioactive medicinal herb, as well as its potential influence on the cytotoxic profile of DOX in naïve (MCF-7) and doxorubicin-resistant (MCF-7ADR) breast cancer cells. The literature reported some in vivo and in vitro studies of anticancer activity of P. niruri in various types of malignancies57. To the best of our knowledge, this is the initial investigation for the impact of P. niruri on resistant breast cancer cells.
According to our data, all tested extracts, and fractions of P. niruri till did not show any promising cytotoxicity against the breast cancer cells alone under investigation (MCF-7 & MCF-7ADR) except for the methylene chloride fraction (F-3). It showed weak cytotoxicity against MCF-7 cells (IC50 = 97.2 μg/ml) and surprisingly higher activity against the DOX resistant breast cancer cells (MCF-7ADR). These findings were consistent with a few previous reports for the potential anticancer activity of aqueous and methanolic extracts of P. niruri against MCF-7 cells20,58.
However, the promising cytotoxicity of CH2Cl2 fraction against MCF-7ADR cells may be in part due to the reported P-glycoprotein (Pgp) inhibitory activity of Phyllanthus extracts in Pgp overexpressing cell lines59,60. The activity of Pgp is implicated in multidrug resistance (MDR) of breast cancer and MCF-7ADR cell line is the commonly used breast cancer cell model for the study of MDR relayed resistance61,62.
The discrepancy in the effectiveness of P. niruri different extracts against cancer cells may be attributed to the difference in the present of bioactive substances. Therefore, untargeted metabolite profiling of the MeOH extract and the active cytotoxic CH2Cl2 fraction was performed using LC-DAD-QToF. The LC–MS profiling of the active CH2Cl2 revealed the presence of main compounds such as the ellagitannin phyllanthussiin U (observed in positive mode) and flavonoids galangin-8-sulfonic acid and niruriflavone (marked in negative ion mode), in addition to six lignans nirtetralin, phyltetralin, phyllanthin, hypophyllanthin, niranthin and isolintetralin. These lignans are well known for their anticancer activities63,64 that enrich the active CH2Cl2 fraction as seen in LC–MS.
In MCF-7 cells, combining DOX with P. niruri extracts (except for CH2Cl2 fraction) at a sub-cytotoxic concentration unexpectedly resulted in an extensively negative modification of doxorubicin’s activity. These combinations not only didn’t show improvement in DOX-induced viability inhibition but additionally showed an increase in the IC50 values of DOX in combinations to nearly ten times of DOX alone, suggesting an antagonistic type of interaction. This makes a lot of sense if we consider the strong antioxidant properties (due to its phenolic content) of this fraction and the mode of action for DOX which involves the generation of reactive oxygen species. Also, this finding is in line with previous observations for the combinations that sometimes resulted in antagonistic effects suggesting that combination at different dose levels was diverse and specific to each combination and cell line65. The involved mechanisms in these interactions between DOX and P. niruri warrants further evaluation.
Surprisingly, in MCF-7ADR, the opposite occurred for most combinations with DOX, which showed potentiation of activity manifested by significant decrease in the IC50 values of DOX in combinations. These findings suggested that P. nurini derivatives could be effective MDR reversal agents, and accordingly they will synergize the activity of most of the standard chemotherapeutics such as DOX66. Another important observation, CH2Cl2 fraction showed the maximal DOX activity potentiation in both cell lines.
According to our observation, P. nurini CH2Cl2 fraction alone showed moderate anti-proliferative effects. However, it enhanced the cytotoxic profile of DOX by 4 folds and 40 folds against MCF-7 and MCF-7ADR, respectively. Several studies described the significance of Phyllanthus alone as an anti-cancer agent against distinct types of malignancy20,22. In addition, several publications including ours showed promising chemomodulatory activities of Phyllanthus to several chemotherapeutics in various types of malignancy65,67.
Combining the results of the two cell lines, Fr-3 had the lowest IC50 values against naïve and resistant MCF-7 cells. Thus, it was selected for further TLC fractionation and investigation for their cytotoxicity and chemomodulatory potential against breast cancer cells. In the same manner, subfractions behave, showing more cytotoxic activity as well as chemomodulatory effect against MCF-7ADR. In addition, TLC fractionation showed that B2 subfraction (Rf 0.2–0.35) was the most potent one in terms of cytotoxicity when combined with DOX.
Some studies suggested that the production of reactive oxygen species (ROS) after DOX treatment is responsible for the cytotoxicity in cancer cells, which could stimulate the production of antioxidant enzymes, like GSH, as a protective defense mechanism of cancer cells making it resistant to oxidative damage. Similarly, greater sensitivity to DOX was observed in breast cancer when the GSH levels decreased68,69. Herein, GSH level reduction observed by Phyllanthus niruri may explain the enhanced chemotherapeutic effect of DOX via sensitizing the resistant MCF-7ADR cells70.
This finding supports our hypothesis that combinations of plant extracts and chemotherapeutic agents may allow for a significant reduction in the dosage of the more toxic chemotherapeutic agent while retaining the therapeutic efficacy and minimizing toxicities not only in naïve tumor cells but also and to a greater extent in the resistant tumor cells. Therefore, additional studies are needed for separation of pure compounds and further elucidation of mechanistic studies for the anticancer and chemomodulatory effects in naïve as well as resistant breast cancer cells.
Combined pharmacotherapy is a common approach that would improve the anticancer activities of cytotoxic drugs while lowering chemo-resistance of cancer cells. Accordingly, and to the best of our knowledge, this is the first study considering anticancer properties of P. niruri in resistant breast cancer alone and in combination with DOX. P. niruri is a promising chemomodulatory agent against resistant breast cancer. Hence, future investigations should include its effect in detail on resistant breast cancer and other MDR overexpressing cancer cells.
All data generated or analyzed during this study are included in this published article and its supplementary information file.
Fontana, F., Anselmi, M. & Limonta, P. Molecular mechanisms of cancer drug resistance: emerging biomarkers and promising targets to overcome tumor progression. Cancers (Basel) https://doi.org/10.3390/cancers14071614 (2022).
Ben Toumia, I. et al. A methanol extract of Scabiosa atropurpurea enhances doxorubicin cytotoxicity against resistant colorectal cancer cells in vitro. Molecules https://doi.org/10.3390/molecules25225265 (2020).
Article PubMed PubMed Central Google Scholar
Khasraw, M., Bell, R. & Dang, C. Epirubicin: is it like doxorubicin in breast cancer? A clinical review. Breast 21, 142–149 (2012).
Łukasiewicz, S. et al. Breast cancer—epidemiology, risk factors, classification, prognostic markers, and current treatment strategies: an updated review. Cancers 13, 4287 (2021).
Article PubMed PubMed Central Google Scholar
Liu, Y., Wang, M., Liu, W., Jing, J. & Ma, H. Olaparib and doxorubicin co-loaded polypeptide Nanogel for enhanced breast cancer therapy. Front Bioeng Biotechnol 10, 904344. https://doi.org/10.3389/fbioe.2022.904344 (2022).
Article PubMed PubMed Central Google Scholar
Argenziano, M. et al. Improvement in the anti-tumor efficacy of doxorubicin nanosponges in in vitro and in mice bearing breast tumor models. Cancers Basel https://doi.org/10.3390/cancers12010162 (2020).
Article PubMed PubMed Central Google Scholar
Ibrahim-Fouad, G. & Ahmed, K. A. Curcumin ameliorates doxorubicin-induced cardiotoxicity and hepatotoxicity via suppressing oxidative stress and modulating iNOS, NF-κB, and TNF-α in rats. Cardiovasc Toxicol. 22, 152–166 (2022).
Article CAS PubMed Google Scholar
Okpara, E. S. et al. Molecular mechanisms associated with the chemoprotective role of protocatechuic acid and its potential benefits in the amelioration of doxorubicin-induced cardiotoxicity: a review. Toxicol. Rep. (2022).
Hadi, N. A., Mahmood, R. I. & Al-Saffar, A. Z. Evaluation of antioxidant enzyme activity in doxorubicin treated breast cancer patients in Iraq: a molecular and cytotoxic study. Gene Rep. 24, 101285 (2021).
Cheraghi, O. et al. The effect of Nrf2 deletion on the proteomic signature in a human colorectal cancer cell line. BMC Cancer 22, 1–16 (2022).
Henidi, H. A., Al-Abbasi, F. A., El-Moselhy, M. A., El-Bassossy, H. M. & Al-Abd, A. M. Despite blocking doxorubicin-induced vascular damage, quercetin ameliorates its antibreast cancer activity. Oxid Med Cell Longev 2020, 8157640. https://doi.org/10.1155/2020/8157640 (2020).
Article CAS PubMed PubMed Central Google Scholar
Shrihastini, V. et al. Plant derived bioactive compounds, their anti-cancer effects and in silico approaches as an alternative target treatment strategy for breast cancer: an updated overview. Cancers Basel https://doi.org/10.3390/cancers13246222 (2021).
Article PubMed PubMed Central Google Scholar
Nisar, M. F. et al. Chemical components and biological activities of the genus Phyllanthus: a review of the recent literature. Molecules https://doi.org/10.3390/molecules23102567 (2018).
Article PubMed PubMed Central Google Scholar
Manjrekar, A. P. et al. Effect of Phyllanthus niruri Linn. treatment on liver, kidney and testes in CCl4 induced hepatotoxic rats. Indian J Exp Biol 46, 514–520 (2008).
Najari Beidokhti, M. et al. Investigation of antidiabetic potential of Phyllanthus niruri L. using assays for alpha-glucosidase, muscle glucose transport, liver glucose production, and adipogenesis. Biochem Biophys Res Commun 493, 869–874. https://doi.org/10.1016/j.bbrc.2017.09.080 (2017).
Article CAS PubMed Google Scholar
Al Zarzour, R. H. et al. Phyllanthus niruri standardized extract alleviates the progression of non-alcoholic fatty liver disease and decreases atherosclerotic risk in sprague-dawley rats. Nutrients 9, 55. https://doi.org/10.3390/nu9070766 (2017).
Marhaeny, H. D., Widyawaruyanti, A., Widiandani, T., Fuad Hafid, A. & Wahyuni, T. S. Phyllanthin and hypophyllanthin, the isolated compounds of Phyllanthus niruri inhibit protein receptor of corona virus (COVID-19) through in silico approach. J Basic Clin Physiol Pharmacol 32, 809–815. https://doi.org/10.1515/jbcpp-2020-0473 (2021).
Article CAS PubMed Google Scholar
Paul, S., Patra, D. & Kundu, R. Lignan enriched fraction (LRF) of Phyllanthus amarus promotes apoptotic cell death in human cervical cancer cells in vitro. Sci Rep 9, 14950. https://doi.org/10.1038/s41598-019-51480-7 (2019).
Article ADS CAS PubMed PubMed Central Google Scholar
Young, A. N. et al. Phyllanthusmin derivatives induce apoptosis and reduce tumor burden in high-grade serous ovarian cancer by late-stage autophagy inhibition. Mol Cancer Ther 17, 2123–2135. https://doi.org/10.1158/1535-7163.MCT-17-1195 (2018).
Article CAS PubMed PubMed Central Google Scholar
Lee, S. H., Jaganath, I. B., Wang, S. M. & Sekaran, S. D. Antimetastatic effects of Phyllanthus on human lung (A549) and breast (MCF-7) cancer cell lines. PLoS ONE 6, e20994. https://doi.org/10.1371/journal.pone.0020994 (2011).
Article ADS CAS PubMed PubMed Central Google Scholar
Ramadhani, A. H. et al. Suppression of hypoxia and inflammatory pathways by Phyllanthus niruri extract inhibits angiogenesis in DMBA-induced breast cancer mice. Res Pharm Sci 16, 217–226. https://doi.org/10.4103/1735-5362.310528 (2021).
Article PubMed PubMed Central Google Scholar
Tang, Y. Q., Jaganath, I., Manikam, R. & Sekaran, S. D. Phyllanthus suppresses prostate cancer cell, PC-3, proliferation and induces apoptosis through multiple signalling pathways (MAPKs, PI3K/Akt, NFkappaB, and hypoxia). Evid Based Complement Alternat Med 609, 55. https://doi.org/10.1155/2013/609581 (2013).
de Araujo Junior, R. F. et al. A dry extract of Phyllanthus niruri protects normal cells and induces apoptosis in human liver carcinoma cells. Exp Biol Med Maywood 237, 1281–1288. https://doi.org/10.1258/ebm.2012.012130 (2012).
Article CAS PubMed Google Scholar
Saahene, R. O. et al. A review: mechanism of Phyllanthus urinaria in cancers-NF-kappaB, P13K/AKT, and MAPKs signaling activation. Evid Based Complement Alternat Med 2021, 4514342. https://doi.org/10.1155/2021/4514342 (2021).
Article PubMed PubMed Central Google Scholar
Meselhy, M. R. et al. Preparation of Lignan-rich extract from the aerial parts of Phyllanthus niruri using nonconventional methods. Molecules 25, 1179 (2020).
Article CAS PubMed PubMed Central Google Scholar
Skehan, P. et al. New colorimetric cytotoxicity assay for anticancer-drug screening. JNCI: J. Natl. Cancer Inst. 82, 1107–1112 (1990).
Eldeeb, M. et al. Anticancer effects with molecular docking confirmation of newly synthesized Isatin sulfonamide molecular hybrid derivatives against hepatic cancer cell lines. Biomedicines 10, 722 (2022).
Article CAS PubMed PubMed Central Google Scholar
Allam, R. M. et al. Fingolimod interrupts the cross talk between estrogen metabolism and sphingolipid metabolism within prostate cancer cells. Toxicol Lett 291, 77–85. https://doi.org/10.1016/j.toxlet.2018.04.008 (2018).
Article CAS PubMed Google Scholar
Chou, T. C. & Talalay, P. Quantitative analysis of dose-effect relationships: the combined effects of multiple drugs or enzyme inhibitors. Adv Enzyme Regul 22, 27–55. https://doi.org/10.1016/0065-2571(84)90007-4 (1984).
Article CAS PubMed Google Scholar
Buckingham, J. Dictionary of natural products. (CRC Press, 1993).
Kumar, B., Kumar, S. & Madhusudanan, K. Phytochemistry of Plants of Genus Phyllanthus. (CRC Press, 2020).
Kumar, S., Singh, A. & Kumar, B. Identification and characterization of phenolics and terpenoids from ethanolic extracts of Phyllanthus species by HPLC-ESI-QTOF-MS/MS. J. Pharmaceutical Anal. 7, 214–222 (2017).
Yang, B., Kortesniemi, M., Liu, P., Karonen, M. & Salminen, J.-P. Analysis of hydrolyzable tannins and other phenolic compounds in emblic leafflower (Phyllanthus emblica L.) fruits by high performance liquid chromatography–electrospray ionization mass spectrometry. J. Agric. Food Chem. 60, 8672–8683 (2012).
Alam, M. B. et al. High resolution mass spectroscopy-based secondary metabolite profiling of Nymphaea nouchali (Burm. f) stem attenuates oxidative stress via regulation of MAPK/Nrf2/HO-1/ROS pathway. Antioxidants 10, 719 (2021).
Tong, S.-R., Lee, T.-H., Cheong, S.-K. & Lim, Y.-M. Untargeted metabolite profiling on the water-soluble metabolites of edible bird’s nest through liquid chromatography-mass spectrometry. Veterinary World 13, 304 (2020).
Article CAS PubMed PubMed Central Google Scholar
Zhong, B. et al. Lc-esi-qtof-ms/ms characterization of seaweed phenolics and their antioxidant potential. Mar. Drugs 18, 331 (2020).
Article CAS PubMed PubMed Central Google Scholar
Peron, G. et al. Antiadhesive activity and metabolomics analysis of rat urine after cranberry (Vaccinium macrocarpon Aiton) administration. J. Agric. Food Chem. 65, 5657–5667 (2017).
Article CAS PubMed Google Scholar
Correia, M. S., Ballet, C., Meistermann, H., Conway, L. P. & Globisch, D. Comprehensive kinetic and substrate specificity analysis of an arylsulfatase from Helix pomatia using mass spectrometry. Bioorg. Med. Chem. 27, 955–962 (2019).
Article CAS PubMed Google Scholar
Kačar, D. et al. Identification of trans-AT polyketide clusters in two marine bacteria reveals cryptic similarities between distinct symbiosis factors. Environ. Microbiol. 23, 2509–2521 (2021).
Xiao, S. et al. Deciphering the differentiations of traditional Chinese medicine analogous formulae by parallel liquid chromatography-mass spectrometry coupled with microplate-based assays. Anal. Methods 6, 9283–9290 (2014).
Powell, R. G., Smith, C. R. Jr. & Weisleder, D. Sesbanimide A and related tumor inhibitors from Sesbania drummondii: structure and chemistry. Phytochemistry 23, 2789–2796 (1984).
Ruiz, A. et al. Isolation and structural elucidation of anthocyanidin 3, 7-β-O-diglucosides and caffeoyl-glucaric acids from calafate berries. J. Agric. Food Chem. 62, 6918–6925 (2014).
Article CAS PubMed Google Scholar
Redeuil, K. et al. Identification of novel circulating coffee metabolites in human plasma by liquid chromatography–mass spectrometry. J. Chromatogr. A 1218, 4678–4688 (2011).
Article CAS PubMed Google Scholar
Farrell, T., Poquet, L., Dionisi, F., Barron, D. & Williamson, G. Characterization of hydroxycinnamic acid glucuronide and sulfate conjugates by HPLC–DAD–MS2: enhancing chromatographic quantification and application in Caco-2 cell metabolism. J. Pharm. Biomed. Anal. 55, 1245–1254 (2011).
Article CAS PubMed Google Scholar
Sasot, G. et al. Identification of phenolic metabolites in human urine after the intake of a functional food made from grape extract by a high resolution LTQ-Orbitrap-MS approach. Food Res. Int. 100, 435–444 (2017).
Article CAS PubMed Google Scholar
Fernández-Poyatos, M. D. P., Ruiz-Medina, A., Zengin, G. & Llorent-Martínez, E. J. Phenolic characterization, antioxidant activity, and enzyme inhibitory properties of Berberis thunbergii DC. leaves: a valuable source of phenolic acids. Molecules 24, 4171 (2019).
Article PubMed PubMed Central Google Scholar
Sousa, A. et al. UPLC-QTOF-MSE-based chemometric approach driving the choice of the best extraction process for Phyllanthus niruri. Sep. Sci. Technol. 52, 1696–1706 (2017).
Huang , Y.-L. , Chen , C.-C. , Hsu , F.-L.& Chen , C.-F.Tannins, flavonol sulfonates, and a Norlignan from Phyllanthus virgatus.J. Nat.Prod.61, 1194–1197 (1998).
Article CAS PubMed Google Scholar
Guo, J. et al. Comparison of two exploratory data analysis methods for classification of Phyllanthus chemical fingerprint: unsupervised vs. supervised pattern recognition technologies. Anal. Bioanal. Chem. 407, 1389–1401 (2015).
da Fontoura Sprenger, R. & Cass, Q. B. Characterization of four Phyllanthus species using liquid chromatography coupled to tandem mass spectrometry. J. Chromatography A 1291, 97–103 (2013).
de Almeida, R. T. R. et al. Exploring the rumen fluid metabolome using liquid chromatography-high-resolution mass spectrometry and Molecular Networking. Sci. Rep. 8, 1–8 (2018).
Article ADS CAS Google Scholar
Romero, N. et al. In vitro anthelmintic evaluation of Gliricidia sepium, Leucaena leucocephala, and Pithecellobium dulce: fingerprint analysis of extracts by UHPLC-orbitrap mass spectrometry. Molecules 25, 3002 (2020).
Article CAS PubMed PubMed Central Google Scholar
Barrios, C. H. Global challenges in breast cancer detection and treatment. Breast 62(Suppl 1), S3–S6. https://doi.org/10.1016/j.breast.2022.02.003 (2022).
Article PubMed PubMed Central Google Scholar
Mahmood, R. I., Abbass, A. K., Razali, N., Al-Saffar, A. Z. & Al-Obaidi, J. R. Protein profile of MCF-7 breast cancer cell line treated with lectin delivered by CaCO3NPs revealed changes in molecular chaperones, cytoskeleton, and membrane-associated proteins. Int. J. Biol. Macromol. 184, 636–647 (2021).
Article CAS PubMed Google Scholar
Howard, G. R., Jost, T. A., Yankeelov, T. E. & Brock, A. Quantification of long-term doxorubicin response dynamics in breast cancer cell lines to direct treatment schedules. PLoS Comput Biol 18, e1009104, doi:https://doi.org/10.1371/journal.pcbi.1009104 (2022).
Ali Abdalla, Y. O. et al. Natural products for cancer therapy: a review of their mechanism of actions and toxicity in the past decade. J Trop Med 2022, 5794350. https://doi.org/10.1155/2022/5794350 (2022).
Article PubMed PubMed Central Google Scholar
Kalimuthu, A. K. et al. In silico, in vitro screening of antioxidant and anticancer potentials of bioactive secondary metabolites from an endophytic fungus (Curvularia sp.) from Phyllanthus niruri L. Environ Sci Pollut Res Int, doi:https://doi.org/10.1007/s11356-022-19249-0 (2022).
Lee, S. H., Jaganath, I. B., Atiya, N., Manikam, R. & Sekaran, S. D. Suppression of ERK1/2 and hypoxia pathways by four Phyllanthus species inhibits metastasis of human breast cancer cells. J Food Drug Anal 24, 855–865. https://doi.org/10.1016/j.jfda.2016.03.010 (2016).
Article CAS PubMed PubMed Central Google Scholar
Patel, J. R., Tripathi, P., Sharma, V., Chauhan, N. S. & Dixit, V. K. Phyllanthus amarus: ethnomedicinal uses, phytochemistry and pharmacology: a review. J Ethnopharmacol 138, 286–313. https://doi.org/10.1016/j.jep.2011.09.040 (2011).
Article CAS PubMed Google Scholar
Kassuya , CA , Leite , DF , de Melo , LV , Rehder , VL & Calixto , JB Anti-inflammatory properties of extracts, fractions and lignans isolated from Phyllanthus amarus .Plant Med 71, 721–726.https://doi.org/10.1055/s-2005-871258 (2005).
Article CAS PubMed Google Scholar
Chen, C. H. et al. Overcoming multidrug resistance of breast cancer cells by the micellar doxorubicin nanoparticles of mPEG-PCL-graft-cellulose. J Nanosci Nanotechnol 11, 53–60. https://doi.org/10.1166/jnn.2011.3102 (2011).
Article CAS PubMed Google Scholar
Howard, G. R., Johnson, K. E., Rodriguez Ayala, A., Yankeelov, T. E. & Brock, A. A multi-state model of chemoresistance to characterize phenotypic dynamics in breast cancer. Sci Rep 8, 12058. https://doi.org/10.1038/s41598-018-30467-w (2018).
Article ADS CAS PubMed PubMed Central Google Scholar
Ooi, K. L., Loh, S. I., Sattar, M. A., Muhammad, T. S. T. & Sulaiman, S. F. Cytotoxic, caspase-3 induction and in vivo hepatoprotective effects of phyllanthin, a major constituent of Phyllanthus niruri. J. Funct. Foods 14, 236–243 (2015).
Sujatha, R., Norhanom, A. W., Nurhayati, Z. A. & Sugumaran, M. Cytotoxicity evaluation of five selected Malaysian Phyllanthaceae species on various human cancer cell lines. Journal of Medicinal Plants Research 5, 2267–2273 (2011).
Pinmai, K., Chunlaratthanabhorn, S., Ngamkitidechakul, C., Soonthornchareon, N. & Hahnvajanawong, C. Synergistic growth inhibitory effects of Phyllanthus emblica and Terminalia bellerica extracts with conventional cytotoxic agents: doxorubicin and cisplatin against human hepatocellular carcinoma and lung cancer cells. World J Gastroenterol 14, 1491–1497. https://doi.org/10.3748/wjg.14.1491 (2008).
Article CAS PubMed PubMed Central Google Scholar
Leite, D. F. et al. The cytotoxic effect and the multidrug resistance reversing action of lignans from Phyllanthus amarus. Planta Med 72, 1353–1358. https://doi.org/10.1055/s-2006-951708 (2006).
Article CAS PubMed Google Scholar
Guo, J. R. et al. Effect of Phyllanthus amarus extract on 5-fluorouracil-induced perturbations in ribonucleotide and deoxyribonucleotide pools in HepG2 cell line. Molecules 21, doi:https://doi.org/10.3390/molecules21091254 (2016).
Leonel, C. et al. Expression of glutathione, glutathione peroxidase and glutathione S-transferase pi in canine mammary tumors. BMC Vet. Res. 10, 1–10 (2014).
Salama, M. M., Zaghloul, R. A., Khalil, R. M. & El-Shishtawy, M. M. Sitagliptin potentiates the anti-neoplastic activity of doxorubicin in experimentally-induced mammary adenocarcinoma in mice: implication of oxidative stress, inflammation, angiogenesis, and apoptosis. Sci. Pharm. 90, 42 (2022).
Prasad, N. R., Muthusamy, G., Shanmugam, M. & Ambudkar, S. V. South Asian medicinal compounds as modulators of resistance to chemotherapy and radiotherapy. Cancers 8, 32 (2016).
Article CAS PubMed PubMed Central Google Scholar
This research was made possible as part of the Ministry of Agriculture Malaysia initiative under the New Key Economic Areas Entry Point Project on High Value Herbals awarded to Natural Wellness Biotech (M) Sdn Bhd. The authors express their gratitude and appreciation for the trust and opportunity given.
Open access funding provided by The Science, Technology & Innovation Funding Authority (STDF) in cooperation with The Egyptian Knowledge Bank (EKB).
Pharmacognosy Department, Faculty of Pharmacy, Cairo University, Kasr El Aini St., Cairo, 11562, Egypt
Ola E. Abdel-Sattar, Ali El-Halawany, Essam Abdel-Sattar & Meselhy R. Meselhy
Pharmacology Department, Medical and Clinical Research Institute, National Research Centre, Dokki, Cairo, 12622, Egypt
Rasha Mosa Allam & Ahmed M. Al-Abd
Biomedical Research Division, NAWAH Scientific, Mokattam, Cairo, Egypt
National Center for Natural Products Research, School of Pharmacy, University of Mississippi, University, MS, 38677, USA
Bharathi Avula, Kumar Katragunta & Ikhlas A. Khan
Division of Pharmacognosy, Department of BioMolecular Sciences, School of Pharmacy, University of Mississippi, University, MS, 38677, USA
Department of Molecular Biology, Genetic Engineering and Biotechnology Research Institute (GEBRI), University of Sadat City (USC), Sadat City, 32958, Egypt
School of Pharmaceutical Sciences, Universiti Sains Malaysia, 11700, Gelugor, Penang, Malaysia
You can also search for this author in PubMed Google Scholar
You can also search for this author in PubMed Google Scholar
You can also search for this author in PubMed Google Scholar
You can also search for this author in PubMed Google Scholar
You can also search for this author in PubMed Google Scholar
You can also search for this author in PubMed Google Scholar
You can also search for this author in PubMed Google Scholar
You can also search for this author in PubMed Google Scholar
You can also search for this author in PubMed Google Scholar
You can also search for this author in PubMed Google Scholar
You can also search for this author in PubMed Google Scholar
O.E.A.: Methodology, Investigation, Data curation. R.M.A.: Conceptualization, Supervision, Methodology, Investigation, Writing-Original draft preparation, Writing-Reviewing, and Editing. A.M.A: Conceptualization, Writing-Original draft preparation, Writing-Reviewing, and Editing. A.E.: Conceptualization, Supervision, Methodology, Validation, Investigation, Writing-Original draft preparation, Writing-Reviewing, and Editing. B.A.: Conceptualization, Methodology, Data Analysis, Writing-Original draft preparation, Writing-Reviewing, and Editing. K.K.: Methodology, Investigation, Data Analysis, Writing-Reviewing, and Editing. I.A.K.: Conceptualization, Visualization, Writing-Reviewing, and Editing. E.A.: Conceptualization, Original draft preparation, Writing-Reviewing, and Editing. M.R.M.: Conceptualization, Supervision, Original draft preparation, Writing- Reviewing and Editing. A.M.E.: Conceptualization and Writing-Reviewing and Editing S.O.M.: Conceptualization, Supplying plant material, Editing.
The authors declare that they have no competing interests.
Springer Nature remains neutral with regard to jurisdictional claims in published maps and institutional affiliations.
Open Access This article is licensed under a Creative Commons Attribution 4.0 International License, which permits use, sharing, adaptation, distribution and reproduction in any medium or format, as long as you give appropriate credit to the original author(s) and the source, provide a link to the Creative Commons licence, and indicate if changes were made. The images or other third party material in this article are included in the article's Creative Commons licence, unless indicated otherwise in a credit line to the material. If material is not included in the article's Creative Commons licence and your intended use is not permitted by statutory regulation or exceeds the permitted use, you will need to obtain permission directly from the copyright holder. To view a copy of this licence, visit http://creativecommons.org/licenses/by/4.0/.
Abdel-Sattar, O.E., Allam, R.M., Al-Abd, A.M. et al. Cytotoxic and chemomodulatory effects of Phyllanthus niruri in MCF-7 and MCF-7ADR breast cancer cells. Sci Rep 13, 2683 (2023). https://doi.org/10.1038/s41598-023-29566-0
DOI: https://doi.org/10.1038/s41598-023-29566-0
Anyone you share the following link with will be able to read this content:
Sorry, a shareable link is not currently available for this article.
Provided by the Springer Nature SharedIt content-sharing initiative
By submitting a comment you agree to abide by our Terms and Community Guidelines. If you find something abusive or that does not comply with our terms or guidelines please flag it as inappropriate.
Scientific Reports (Sci Rep) ISSN 2045-2322 (online)
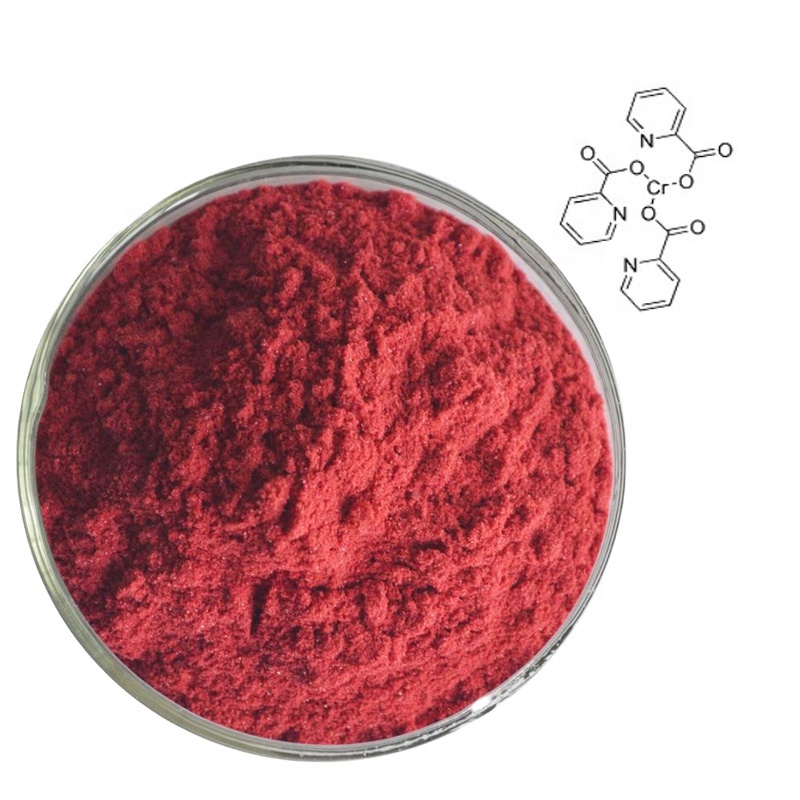
Elderberry Extract Sign up for the Nature Briefing: Cancer newsletter — what matters in cancer research, free to your inbox weekly.